Author: Ginger Ding
“Reflections on CRISPR’s Potential (Part 1)” explores the bottlenecks and challenges of CRISPR clinical applications and uses the case study of CRISPR Therapeutics and Editas to interpret the corporate strategies of different CRISPR companies.
On October 26, Bayer announced a huge acquisition of Asklepios, which focuses on gene therapy, for US$4 billion. Following the trend of other international pharmaceutical companies such as Novartis, Roche, and Celgene, with this acquisition Bayer has also entered the cell and gene therapy track. Since 2018, there have been more than a dozen mergers and acquisitions of cell and gene therapy companies, and the momentum has not diminished. JW Therapeutics, which focuses on cell therapy, was also listed on the Hong Kong Stock Exchange on November 3.

Gene therapy company acquisitions
Data source: Compilation of public data from MyBioGate
There is no doubt that gene editing is critical to cell and gene therapy. In addition to the well-known CRISPR, what other gene editing technologies are comparable or even potentially better? This article will look at the overall picture of gene editing, answering the following questions: Is CRISPR the king of gene editing? Is there a chance for the previous wave of gene editing such as zinc-finger nuclease to return? What new gene editing technologies are booming?
Ongoing gene editing clinical trials
The first hint of the existence of CRISPR-Cas came in 1987 in Japan when an unusual repetitive sequence was discovered in the genome of the Escherichia coli bacteria. However, it was not used in gene editing until 2012. In contrast, zinc-finger nuclease (ZFN) technology, the originator of gene editing technology, was developed as early as the 1990s. It was followed by transcriptional activator-like effector nuclease (TALEN) in 2009.
However, latecomers turned around and currently dominate the gene editing market. CRISPR has quickly become the most widely used gene editing tool in basic research due to its easy use, faster process, and lower cost than both ZFN and TALEN. Within four years it already entered clinical trials. For example, in 2016, West China Hospital of Sichuan University announced the world’s first human CRISPR gene editing clinical trial (knockout PD-1 of the T cell) to treat patients with metastatic non-small cell lung cancer.
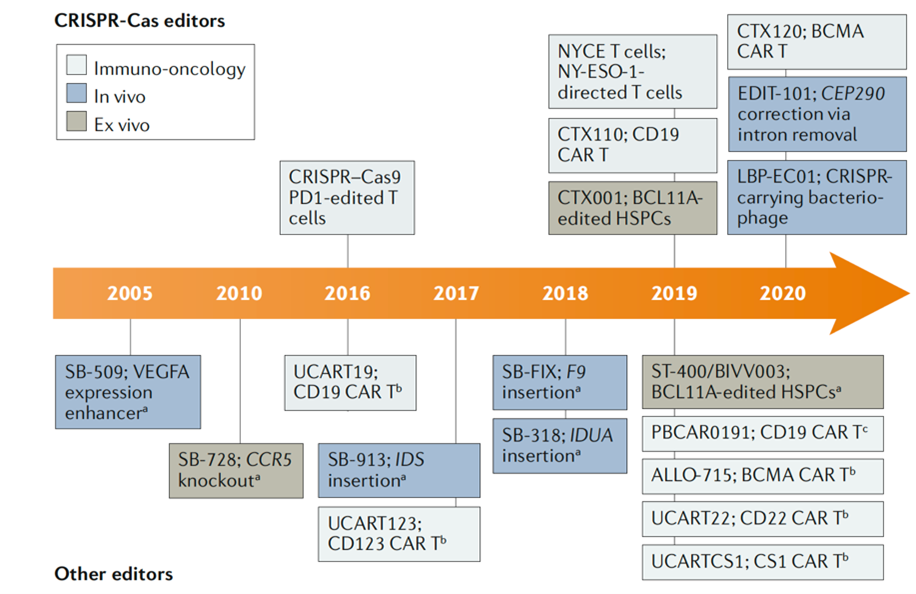
Partial history of gene editing clinical trials
Source: Nat Rev Drug Discov. 2020 Jun; 19(6):367-372.
Meanwhile, in the past year and a half, more than a dozen new gene therapies have entered clinical trials, more than half of which are based on CRISPR【1】. At present, most gene therapies focus on single-gene rare diseases and cancer immunotherapy, but wider applications are also booming.
“As with any new technology, the typical way that that things get rolled out is that drug developers begin with rare and or extremely severe diseases where there aren’t really other options. And the outcomes of the first applications have a very big effect on whether the technology is perceived to be safe or appropriate to be used more widely.”
——Jennifer Doudna, Nobel Prize winner
Compared with in vivo editing, in vitro editing is a low-hanging fruit, due to better and more controllable drug delivery. There are also safety advantages such as reduced immune response as mentioned in “Reflections on CRISPR’s Potential (Part 1)”. There have been multiple clinical trials targeting thalassaemia and other hereditary red blood cell diseases such as Sangamo’s ST-400 and CRISPR Therapeutics and Vertex’s CTX001, which will compete with Bluebird Bio’s approved therapy ZYNTEGLO.

In vitro editing gene therapy clinical trial stages
Source: public information and Nat Rev Drug Discov. 2020 Jun; 19(6):367-372.
In addition, in vitro editing also provides technical support for the development of universal cell therapy. In terms of CAR-T development, China continues to occupy a place in the international arena. Longyao Group’s universal CAR-T therapy CD19UCART and 301 Hospital’s UCART019 have both entered clinical trials. (Note: 301 Hospital is also known as the People’s Liberation Army General Hospital)
It is worth pointing out that, similar to allogeneic CAR-T, Chinese companies and institutions have obvious homogeneity in the selection of targets, mainly concentrated in CD19, and have not yet expanded outwards.

Universal cell therapy clinical trial stages
Source: public information and Nat Rev Drug Discov. 2020 Jun; 19(6):367-372.
One of the reasons why blood diseases have become the early targets for gene editing is that editing can be done in vitro, but achieving in vivo editing is a daunting challenge for gene therapy.
Fortunately, at least seven companies are already working on in vivo editing. Sangamo is the first to do so, striving to surpass other already marketed in vivo gene therapy products, such as Spark’s Luxturna and Avexis’ AVXS-101. Luxturna and AVXS-101 are both based on adeno-associated virus (AAV) for gene delivery, but it is difficult to integrate into the genome. Sangamo’s SB-913 is expected to be directly inserted into cell chromosomal DNA through three vectors.
Although the SB-913 clinical trial did not reach a clinical endpoint in 2019, the data showed that one patient treated with the highest dose had promising initial results, so Sangamo remained optimistic and is actively working to improve the therapy.
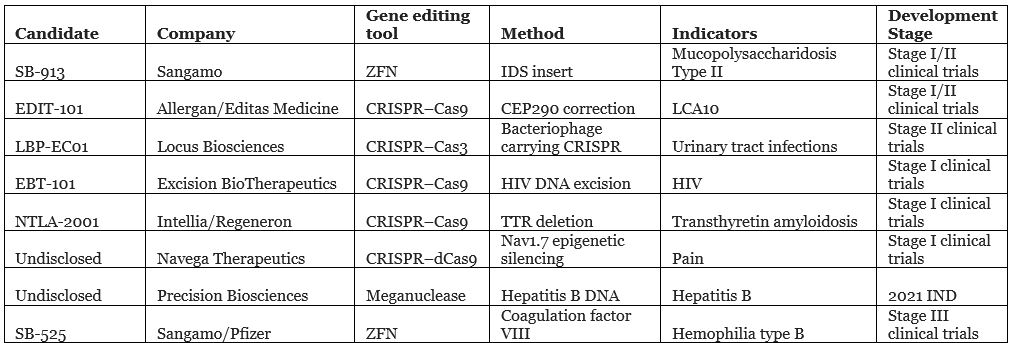
In vivo editing gene therapies clinical trial stages
Source: public information and Nat Rev Drug Discov. 2020 Jun;19(6):367-372.
Comparative analysis of three mainstream gene editing technologies
.

Editing technology comparison
Data source: Compilation of public information by MyBioGate
Based on the clinical list in Part 1, we can see that in addition to Precision Biosciences’ ARC nuclease, CRISPR, ZFN, TALEN, and their upgraded versions (such as Beam Therapeutics’ CRISPR base editor) are still the three giants of gene editing technology.
The biggest advantages of CRISPR are that it does not involve protein engineering, is simple to operate, has the fastest design speed (a few days), low cost (within a few hundred dollars), and can achieve high throughput, so ordinary laboratories can use CRISPR/ Cas9 technology to easily achieve gene editing. With TALEN and ZFN, scientists often have to spend a lot of money and hand over the gene editing work to biotech companies.
“It’s simple enough to deploy that essentially any starting graduate student, with a little knowledge of molecular biology, can use it to introduce changes in cells of interest.”
——Jennifer Doudna, Nobel Prize winner
The above advantages play a decisive role in CRISPR’s popularity. In fact, CRISPR has quickly become widely used in scientific research in laboratories around the world. However, from the perspective of clinical application, a low technical barrier means high competition. In addition, CRISPR has several major limitations that cannot be ignored, including the limitations of PAM sequencing mentioned in “Reflections on CRISPR’s Potential (Part 1)”. Immune responses, off-target effects, potential carcinogenicity, and immunogenicity and drug delivery challenges are further factors to consider.
Unlike the complex dimer structure of ZFN and TALEN, the CRISPR system is a monomer structure that binds through base pairing, so the specificity is relatively low. Of course, scientists have developed various strategies to increase specificity, including the use of enzymes from different bacteria, recombinant Cas9 enzymes, and adjusting secondary structure of the recognition sequence in the guide RNA. This process will be further elaborated in Part 3.
Furthermore, one of the flaws of the Cas enzyme is its large protein size. It is challenging to deliver its genes to cells through vectors such as adeno-associated viruses, which are commonly used in gene therapy.
Unlike CRISPR and TALEN, ZFN is not derived from a bacterial system. Thus, its immunogenicity is predicted to be low and has been verified in humans and multiple biological models such as zebrafish, mice, and others. Its biggest shortcoming is its long design time, but this has been shortened from a few months to two weeks【1】. Because of high technical barriers, Sangamo is the sole leader for ZFN development. Sangamo has been deeply involved for more than 20 years in ZFN and has received support from many international pharmaceutical companies such as Pfizer, Takeda, Sanofi, Novartis, and Biogen.

Sangamo product line
Source: Sangamo public profile
TALEN, as a second-generation gene editing nuclease, can prevent off-target effects to a greater extent than other gene editing technologies. Compared with ZFN, TALEN is simpler to operate, faster to construct, and less expensive. Compared with CRISPR, the assembly of plasmid is more time cosuming and labor-intensive, which leads to higher costs. Companies deploying TALENs mainly include Cellectis and Allogene, while Bluebird Bio took a different approach and synthesized megaTALs integrated with TALEN and meganucleases.

Cost comparison between CRISPR and TALEN
Source: ARK Investment
It can be seen that traditional CRISPR has no absolute advantages other than a lower cost and easy technique. In order to surpass ZFN and TALEN in clinical applications, it is necessary to find a breakthrough in controlling off-target and improving delivery efficiency in these two key directions. Part 3 will take stock of an upgraded version of CRISPR from this perspective.
Gene editing’s rising stars
This section will focus on gene editing platforms that break through the bottlenecks and limitations of traditional technologies.
1. Precision BioSciences (NASDAQ: DTIL): a new generation of gene editing enzymes

In addition to the above-mentioned three giants, ARCUS nuclease, which is affiliated with Precision BioSciences, has emerged on the scene in clinical gene editing therapy.
ARCUS is part of a new generation of genome editing, derived from the natural genome editing enzyme homing endonuclease. The homing endonuclease can recognize up to 40 base pairs, has a small size and unparalleled sequence specificity, and can also achieve custom editing. Precision Biosciences has a complete set of projects for editing, embedding, and deleting DNA. Judging from the initial data, the safety profile and AAV8 delivery efficiency are remarkable.
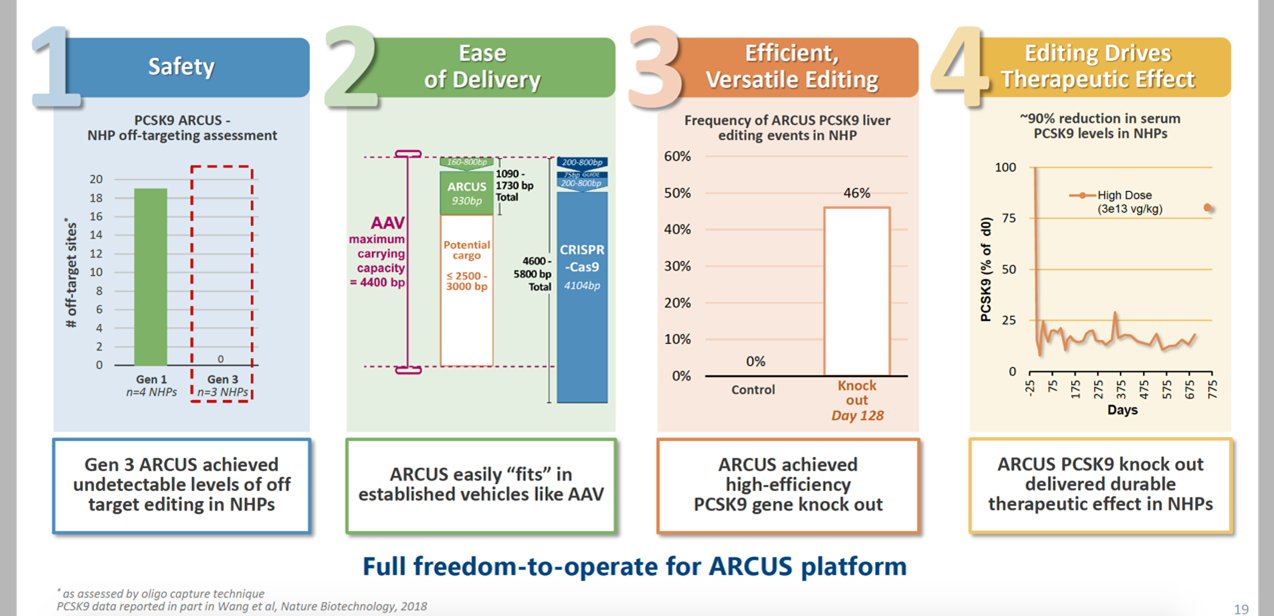
In the process of finding a cure for hepatitis B, Precision BioSciences has made a breakthrough: the preclinical data displayed by the American Society for Genetics and Cellular Therapy (ASGCT) in 2020 shows that the optimized ARCUS can effectively target and degrade cccDNA by 75%, and is accompanied by hepatitis B surface antigen knockdown. Given the recent clinical failure of ABI-H0731 developed by Assembly Biosciences, Precision BioSciences might be the next hope.
- Locus Biosciences: Cas3 targeting bacteria

While many clinical studies use CRISPR-Cas9 to edit the human genome, Locus, led by prestigious investor such as Tencent, is using the unique properties of CRISPR-Cas3 to target and eliminate specific bacterial populations, responding to challenges including broad-spectrum antibiotics and antibiotic resistance selection.
In early 2019, Locus and Johnson & Johnson signed a collaboration agreement for the development, production and commercialization of CRISPR-Cas3. The research on escherichia coli has entered clinical trials.
Antibiotic resistance has become a global public health problem. In the face of this huge market, international pharmaceutical companies have entered, failed, exited, and then returned. In a few cases, even though the developers, for example, Achaogen and Tetraphase, succefully crossed the finish line. Given the super low cost of antibiotics, it is challenging to penetrate the market. Therefore, the commercial value of Locus Biosciences remains to be seen.
3. Arbor Biotechnologies:The smallest Cas enzyme to date

Similar to Beam Therapeutics, another company founded by Zhang Feng, Arbor Biotechnologies also focuses on the development of Cas13.
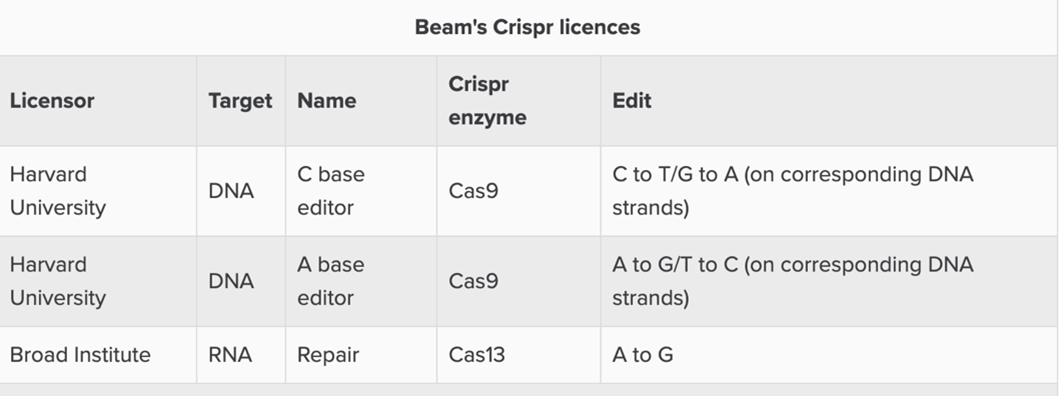
Source: Evaluate
Arbor’s patented Cas13d protein, although it belongs to the Cas13 family, is smaller than other members (about 900 amino acids, based on the average 1kb of 333 amino acids, and about 2.7kb, which is similar to TALEN’s size) This small size means it has greater potential. Arbor was established in 2016 and obtained the Cas13d patent in 2019. There is not much public information thus its potential is difficult to judge for the time being.
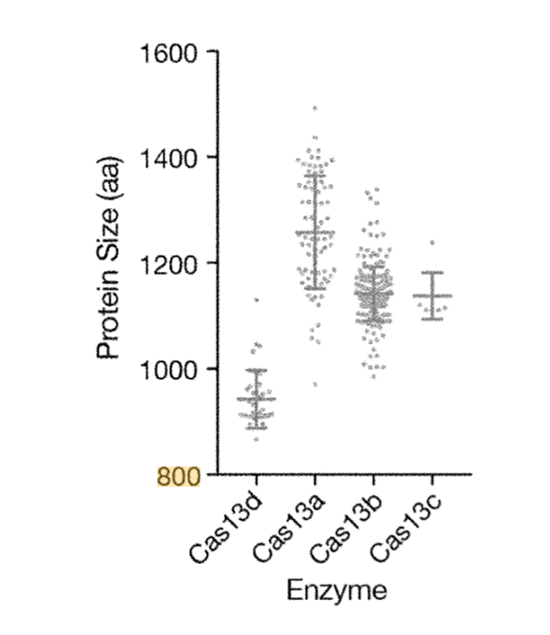
Source: Patent (US10392616B2)
4. Scribe Therapeutics: Focus on the development of new CRISPR molecules
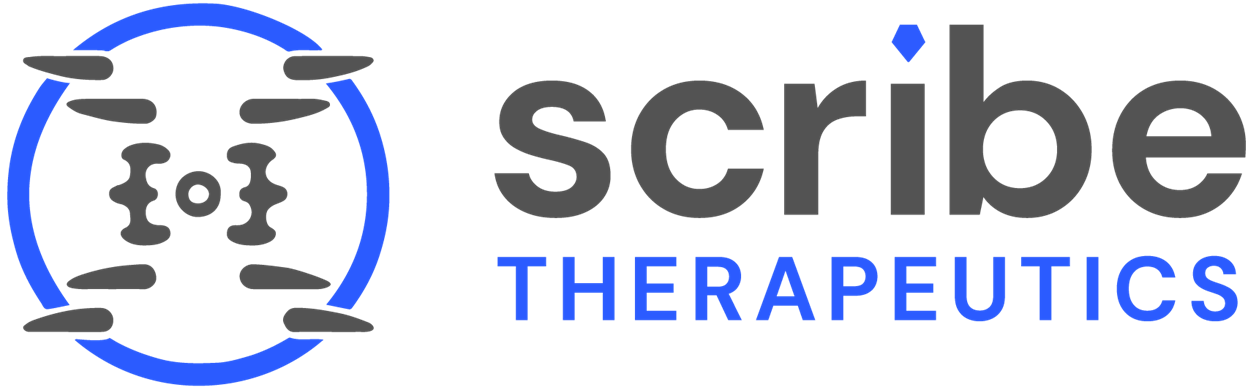
After founding Caribou Biosciences and Mammoth Biosciences, Jennifer Doudna founded Scribe Therapeutics.
Scribe is a new CRISPR molecule that specifically develops therapeutic properties. Scribe’s first technology is called an X-Editing (XE) molecule, which is a highly engineered CRISPR enzyme. Compared with the current CRISPR genome editing technology, it has high specificity and efficient delivery.
On the 6th of 2020, Scribe announced the establishment of a partnership with Biogen to develop methods for the treatment of neurological diseases such as amyotrophic lateral sclerosis. If successful, it could bring more than US$400 million in collaboration benefits.
5. Massachusetts General Hospital: PAMless CRISPR

A major limitation of the application of CRISPR is its reliance on the PAM sequence. In addition to the research of Zhang Feng and David Liu, this year Massachusetts General Hospital has received a lot of attention. This is thanks to the Kleinstiver Lab team.
In March 2020, the Kleinstiver Lab team published a paper entitled “Unconstrained genome targeting with near-PAMless engineered CRISPR-Cas9 variants” in “Science”. Through genetic modification, the paper stated that SpG and SpRY does not require specific PAM sequencing. The Cas9 protein variant that binds and cleaves DNA can target most human genomes without restriction and has single base pair range accuracy【1】.

It is worth noting that apart from Kleinstiver, the inventors of the patents of SpG and SpRY also include J. Keith Joung, one of the founders of Editas.

6. “Jumping Gene”: An upgraded version of CRISPR challenges the “off-target” effect

In 2019, two new kinds of CRISPR were introduced in “Science” and “Nature”. Different from classic CRISPR, these two upgraded versions of CRISPR use a “jumping gene”, also known as a transposon. One of the studies comes from Zhang Feng.
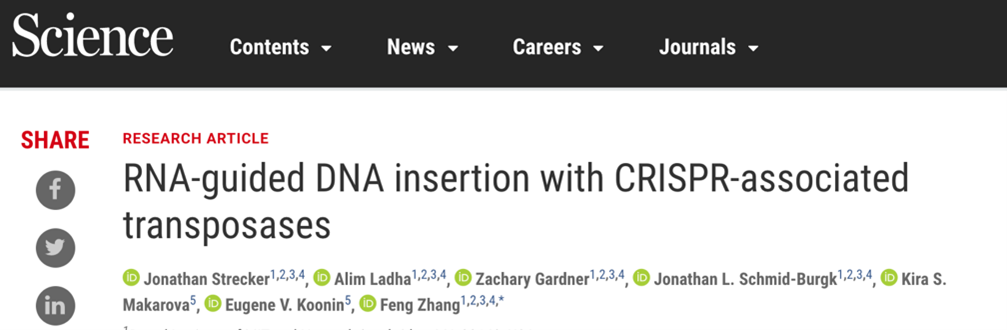
The “jumping gene” is like a “molecular glue”. It is not a simple cutting of double-stranded DNA, and then relies on homologous recombination mechanism to repair the cut site. The new system enables genes to “jump” and integrate them into different sites in the genome and does not involve double-strand breaks or homologous recombination repair, so it can effectively avoid getting off-target.
Zhang Feng’s team used the Tn7 transposon with the Cas12 enzyme of CRISPR. Under the guidance of CRISPR, Tn7 traps the molecular “scissors” and inserts itself into the target genome without cutting the DNA. The success rate of gene insertion is as high as 80%【2】.
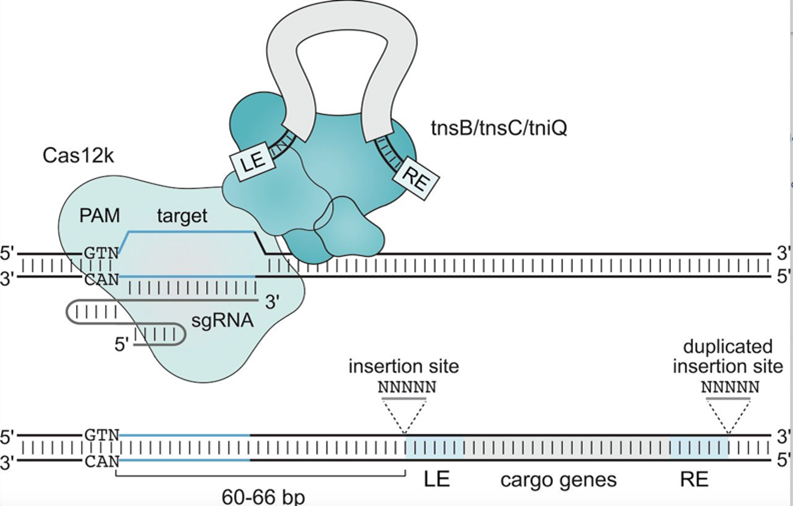
Source: Science. 2020 Jun 5;368(6495):eabb2920.
Compared with the CRISPR system of Zhang Feng’s team, the highlight of Samuel Sternberg’s team is that the insertion system can be inserted randomly in both positive and negative directions. This new technology is called “INTEGRATE”【3】.
Conclusion
A new generation of gene editing tools is emerging. In addition to its own evolution, another challenge that cannot be ignored is effective drug delivery, especially for in vivo editing applications.
The most widely used delivery vector for gene editing is AAV. However, a large number of studies have shown that some patients have pre-existing immunity to AAV vectors. If they receive repeated administrations, patients may also develop AAV immunity【4】. Gene therapy pioneer Jim Wilson once warned of the potential safety hazards caused by AAV. For example, the FDA stopped Solid Biosciences’ SGT-001 clinical development twice due to safety issues.
Compared with the traditional AAV9, the AAV8 currently used by Precision BioSciences and others can be cleared from the blood faster to avoid an immune response. However, a shortcoming of all AAVs is that they tend to be delivered to the liver, muscles and central nervous system, which limits the types of tissues that can be effectively edited.
In addition to AAV, Intellia and partner Regeneron are exploring lipid nanoparticle (LNP) delivery systems. In the past few decades, the FDA and EMA have approved more than a dozen drugs using LNP for delivery, including Onpattro, which wins Alnylam a big success. Carried by LNP, the intravenous Onpattro smoothly enters the liver cells where the target is located along with the blood circulation, silences related genes and removes the pathogenic proteins deposited in the body.
In the long run, as editing applications become more and more complex, multiple cuts at a time are the next goal, so carriers with greater carrying capacity will have more potential.
About the author
Dr. Ginger Ding is the Director of Investment Analysis at MyBioGate and a Consultant for the Texas Medical Center and Columbia University Consulting Club. She has worked at the Howard Hughes Medical Institute and MD Anderson Cancer Center. During that period, she participated in the preclinical research of tumor brain metastasis and received funding from the National Institutes of Health RO1, Susan G. Komen, and Taiho Pharmaceutical.
References
【1】Nat Rev Drug Discov. 2020 Jun;19(6):367-372.
【2】Beyond CRISPR: What’s current and upcoming in genome editing
【3】Science. 2020 Apr 17;368(6488):290-296
【4】Science. 2020 Jun 5;368(6495):eabb2920.
【5】Nature. 2019 Jul;571(7764):219-225
【6】Mol Ther. 2020 Mar 4;28(3):723-746
0 Comments